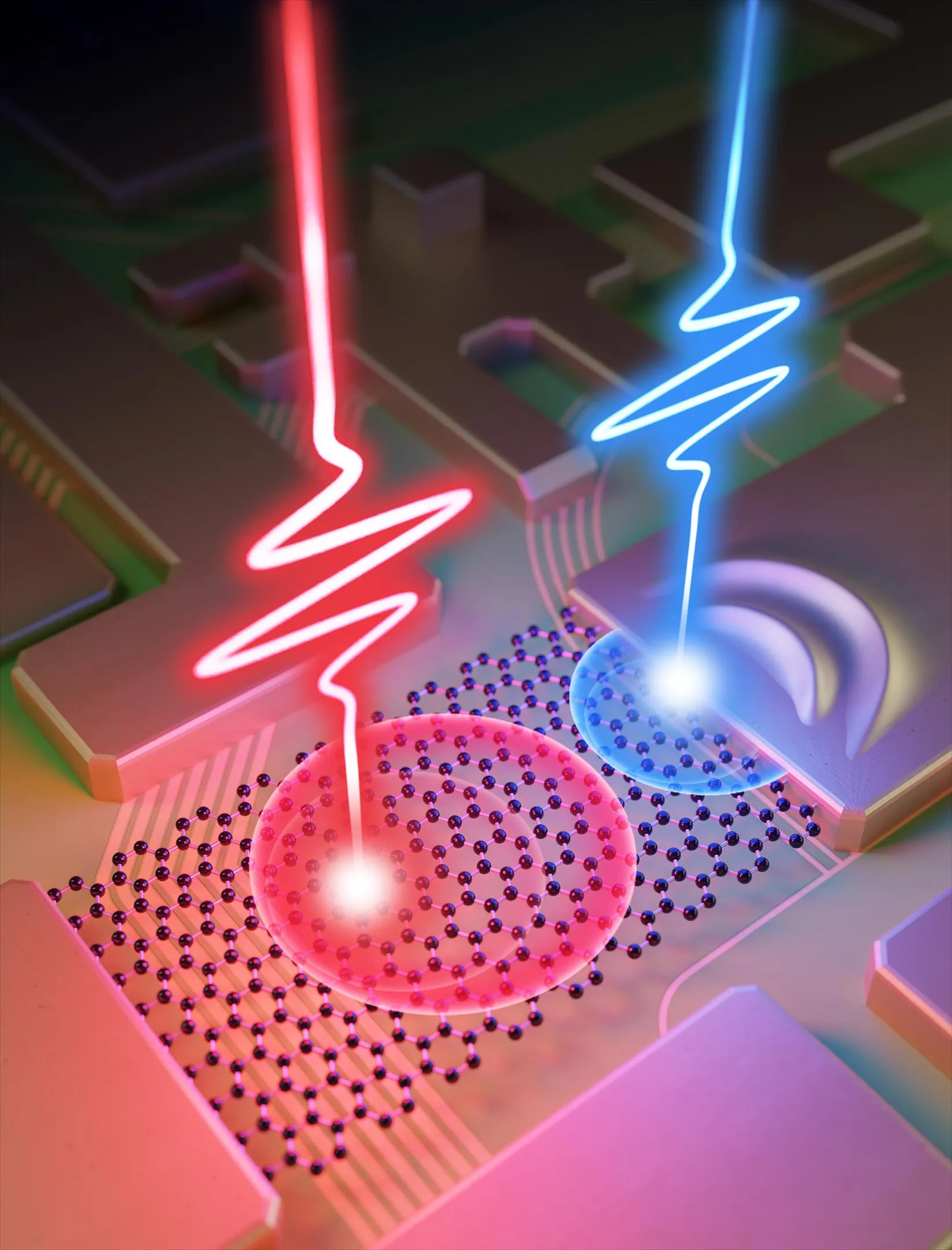
Des impulsions laser synchronisées (rouge et bleu) génèrent une salve de porteurs de charge réels et virtuels dans le graphène, qui sont absorbés par l’or métallique pour produire un courant net. “Nous avons clarifié le rôle des porteurs de charge virtuels et réels dans les courants induits par laser, ce qui a ouvert la voie à la création de portes logiques ultrarapides”, explique Ignacio Franco, professeur associé de chimie et de physique à l’université de Rochester. Crédit : illustration de l’Université de Rochester / Michael Osadciw
Les chercheurs ont fait un pas décisif vers la création d’ordinateurs ultrarapides.
Une quête de longue date pour la science et la technologie a été de créer de l’électronique et du traitement de l’information qui fonctionnent près des échelles de temps les plus rapides permises par les lois de la nature.
Une approche prometteuse pour atteindre cet objectif consiste à utiliser la lumière laser pour guider le mouvement des électrons dans la matière, puis à utiliser ce contrôle pour développer des éléments de circuits électroniques – un concept connu sous le nom d’électronique à ondes lumineuses.
De façon remarquable, les lasers nous permettent actuellement de générer des rafales d’électricité à l’échelle de la femtoseconde, c’est-à-dire en un millionième de milliardième de seconde. Pourtant, notre capacité à traiter l’information à des échelles de temps aussi ultrarapides est restée insaisissable.
“Nous savons maintenant que l’électronique à ondes lumineuses est pratiquement possible.” – Tobias Boolakee
Aujourd’hui, des chercheurs de l’Université de Rochester et de la Friedrich-Alexander-Universität Erlangen-Nürnberg (FAU) ont fait un pas décisif dans cette direction en démontrant une porte logique – la brique de base du calcul et du traitement de l’information – qui fonctionne à l’échelle de la femtoseconde. L’exploit, rapporté le 11 mai dans le journal NatureL’exploit, rapporté le 11 mai dans la revueNature, a été réalisé en exploitant et en contrôlant indépendamment, pour la première fois, les porteurs de charge réels et virtuels qui composent ces rafales d’électricité ultrarapides.
Les progrès des chercheurs ont ouvert la porte au traitement de l’information à la limite du pétahertz, où un quadrillion d’opérations de calcul peuvent être traitées par seconde. C’est presque un million de fois plus rapide que les ordinateurs actuels fonctionnant avec des fréquences d’horloge de l’ordre du gigahertz, où 1 pétahertz correspond à 1 million de gigahertz.
“C’est un excellent exemple de la manière dont la science fondamentale peut conduire à de nouvelles technologies”, déclare Ignacio Franco, professeur associé de chimie et de physique à Rochester qui, en collaboration avec le doctorant Antonio José Garzón-Ramírez ’21 (PhD), a réalisé les études théoriques qui ont conduit à cette découverte.
Les lasers génèrent des rafales d’électricité ultrarapides
Ces dernières années, les scientifiques ont appris à exploiter des impulsions laser de quelques femtosecondes pour générer des bouffées de courant électrique ultrarapides. Cela se fait, par exemple, en illuminant de minuscules graphene-based wires connecting two gold metals. The ultrashort laser pulse sets in motion, or “excites,” the electrons in graphene and, importantly, sends them in a particular direction—thus generating a net electrical current.
Laser pulses can produce electricity far faster than any traditional method—and do so in the absence of applied voltage. Further, the direction and magnitude of the current can be controlled simply by varying the shape of the laser pulse (that is, by changing its phase).
The breakthrough: Harnessing real and virtual charge carriers
The research groups of Franco and of FAU’s Peter Hommelhoff have been working for several years to turn light waves into ultrafast current pulses.
In trying to reconcile the experimental measurements at Erlangen with computational simulations at Rochester, the team had a realization: In gold-graphene-gold junctions, it is possible to generate two flavors—“real” and “virtual”—of the particles carrying the charges that compose these bursts of electricity.
- “Real” charge carriers are electrons excited by light that remain in directional motion even after the laser pulse is turned off.
- “Virtual” charge carriers are electrons that are only set in net directional motion while the laser pulse is on. As such, they are elusive species that only live transiently during illumination.
Because the graphene is connected to gold, both real and virtual charge carriers are absorbed by the metal to produce a net current.
Strikingly, the team discovered that by changing the shape of the laser pulse, they could generate currents where only the real or the virtual charge carriers play a role. In other words, they not only generated two flavors of currents, but they also learned how to control them independently, a finding that drastically augments the elements of design in lightwave electronics.
Logic gates through lasers
Using this augmented control landscape, the team was able to experimentally demonstrate, for the first time, logic gates that operate on a femtosecond timescale.
Logic gates are the basic building blocks needed for computations. They control how incoming information, which takes the form of 0 or 1 (known as bits), is processed. Logic gates require two input signals and yield a logic output.
In the researchers’ experiment, the input signals are the shape or phase of two synchronized laser pulses, each one chosen to only generate a burst of real or virtual charge carriers. Depending on the laser phases used, these two contributions to the currents can either add up or cancel out. The net electrical signal can be assigned logical information 0 or 1, yielding an ultrafast logic gate.
“It will probably be a very long time before this technique can be used in a computer chip, but at least we now know that lightwave electronics is practically possible,” says Tobias Boolakee, who led the experimental efforts as a PhD student at FAU.
“Our results pave the way toward ultrafast electronics and information processing,” says Garzón-Ramírez ’21 (PhD), now a postdoctoral researcher at McGill University.
“What is amazing about this logic gate,” Franco says, “is that the operations are performed not in gigahertz, like in regular computers, but in petahertz, which are one million times faster. This is because of the really short laser pulses used that occur in a millionth of a billionth of a second.”
From fundamentals to applications
This new, potentially transformative technology arose from fundamental studies of how charge can be driven in nanoscale systems with lasers.
“Through fundamental theory and its connection with the experiments, we clarified the role of virtual and real charge carriers in laser-induced currents, and that opened the way to the creation of ultrafast logic gates,” says Franco.
The study represents more than 15 years of research by Franco. In 2007, as a PhD student at the University of Toronto, he devised a method to generate ultrafast electrical currents in molecular wires exposed to femtosecond laser pulses. This initial proposal was later implemented experimentally in 2013 and the detailed mechanism behind the experiments explained by the Franco group in a 2018 study. Since then, there has been what Franco calls “explosive” experimental and theoretical growth in this area.
“This is an area where theory and experiments challenge each other and, in doing so, unveil new fundamental discoveries and promising technologies,” he says.
For more on this research, see Laser Pulses for Ultrafast Signal Processing Could Make Computers 1 Million Times Faster.
Reference: “Light-field control of real and virtual charge carriers” by Tobias Boolakee, Christian Heide, Antonio Garzón-Ramírez, Heiko B. Weber, Ignacio Franco and Peter Hommelhoff, 11 May 2022, Nature.
DOI: 10.1038/s41586-022-04565-9
The Franco Lab is supported through awards from the Chemical Theory and Computations program of the National Science Foundation and the Leonard Mandel Faculty Fellowship at the University of Rochester.